National Center for Advancing Translational Sciences
National Institutes of Health
National Cancer Institute
National Institutes of Health
ortable and wearable consumer electronics for communication, interconnectivity, and information sharing have become firmly woven into the fabric of daily living. These technologies and associated infrastructure have spawned potential for the integration of wearable devices into the cancer-care continuum. Digital biomarkers and digital health approaches are being broadly applied to the multifaceted obstacles cancers present at the levels of prevention, risk, detection, diagnosis, treatment, and survivorship.
Wearables in the Digital Health Ecosystem
The current state of the art for digital health is enabling the generation of novel data types that inform baseline human health and its subsequent deviation as it is perturbed from disease or therapeutic intervention. Digital health technologies incorporate any combination of computing platforms, connectivity, software, or sensors to generate digital biomarkers, such as acquisition of high temporal fidelity (albeit less specific) wearable data outside of the traditional clinical setting whose deviation from an individual’s baseline data stream can be the marker. Coupling of data from robust assays (multimodal) with mHealth-derived PROs and/or retrospective real-world evidence/data with clinical trial data are other examples. For all examples, this requires in silico analysis of data sets with modern analytic methods (e.g., artificial intelligence (AI) and machine learning (ML)) to generate biomarkers; this combination of data analytics and physical hardware offers unique opportunities. As a whole, digital health has become an expansion of precision medicine, in that it enables novel insight of the individual relative to the population and vice versa.
Out-of-clinic monitoring devices have been relegated to this burgeoning area of digital health, in large part due to their inability to meet regulatory metrics for biomarkers by themselves. These devices include wearable technologies and ambient in-home sensors among others. While most of these sensors lack the specificity needed to provide actionable clinical information from measured biometrics on their own, they can generate copious data that in aggregate can indicate a move from the wearer’s personal baseline. For these sensors to consistently generate fit-for-purpose information, they require other on-board computing elements (i.e., cyber-physical systems) or cloud-based informatic and analytic elements to process.
Wearable devices offer new solutions to objectively generate fit-for-purpose information that informs the evaluation of therapeutic interventions. The devices simply require a new approach to measure disease-specific signals compared to more traditional assays, which are restricted to the traditional healthcare setting. Out-of-clinic monitoring extends healthcare to more patients and can add more insight into a patient’s individualized trajectory or disease risk profile. This ability to objectively measure individual set(s) of disease or therapeutic perturbations as high-resolution movies, versus snapshots in time as practiced in contemporary medicine, is a major attribute of digital health.
Moving Wearables into Oncology
Traditionally, oncology has not relied on basic biometrics (e.g., activity or sleep) or vital signs as sole metrics of cancer, at least compared to cardiovascular disease. However, opportunities to leverage current remote monitoring technologies (Grades I and II) offer many benefits to oncology both for clinical research and practice. It is anticipated that the next generation of wearable devices (Grade III – on/in body biofluid analyte sampling tools) will increase specificity for oncological practice with the development of more fit-for-purpose sensors measuring local and systemic biochemistry that comprise digital health signatures.
At present, these technologies offer the ability to measure many analytes (see Table 1) continuously, and some (e.g., implantables and microneedle patches) offer a real-time window into blood plasma (e.g., partitioning is in equilibrium between epidermal interstitial fluid and blood plasma for many biochemical analytes). Going forward, the only question for these tools will be which analytes, or sets of measures, will produce actionable digital phenotypes for clinical use. Thus, finding ways to reliably validate actionable signals from these more complex tools, either individually or in tandem with others (e.g., Grade I/II), will continue to be an area of active investigation.
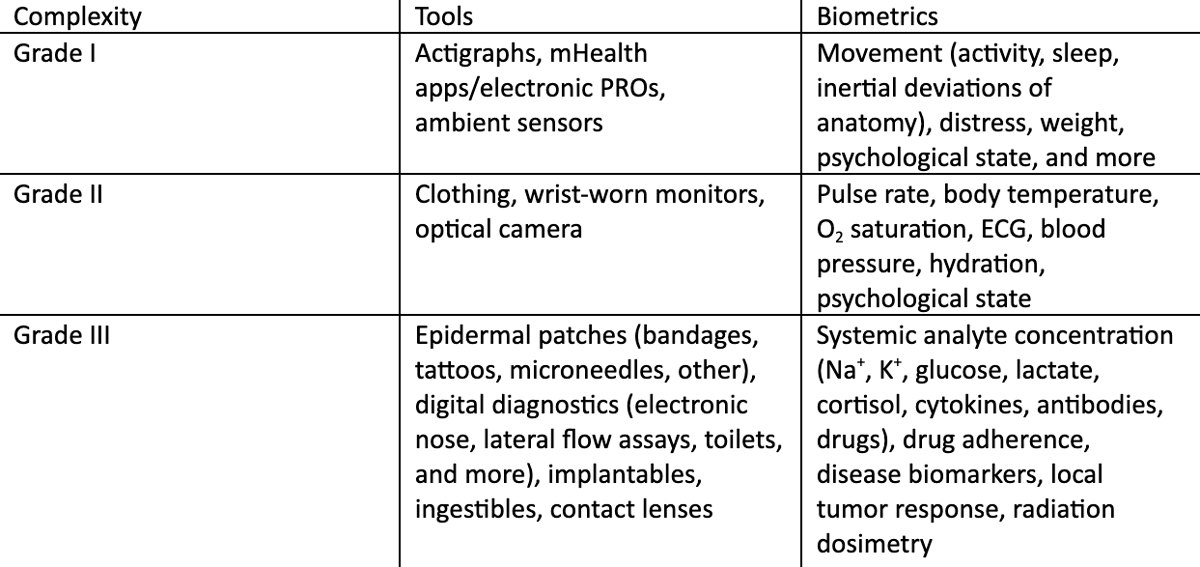
One common metric for oncologists to measure indirect effects of therapy or cancer progression is performance status (assessed in the clinic from answered questions and physical appearance or movement, and scored under ECOG or KS guidelines). Patient performance status is a subjective, yet powerful, tool that is being explored for more objective input from activity monitoring. For example, T. Nilanon and colleagues utilized wearable activity monitors to assess accuracy of performance status scoring and subsequent impact on reducing the risk of unplanned healthcare encounters by patients undergoing cancer chemo- and radiation therapy. Similarly, in a secondary analysis of a clinical trial for measuring latent effects of chemotherapy-induced peripheral neuropathy in cancer survivors with wearables, the researchers developed a digital biomarker for cancer-related fatigue from the same population. Another area to be studied, in terms of how wearables can have clinical impact, is earlier detection, prediction, and/or mitigation of adverse events associated with cancer therapies. For example, two studies have looked at post-treatment frailty (either as a result of therapy or surgical oncology) and developed biomarkers to grade it relative to inertial movement over time within adults and pediatric cancer survivors, respectively. In a similar approach, chemotherapy-induced peripheral neuropathy was graded with respect to the pre-therapy patient baseline. Lastly, wearable sensors are well-suited for longitudinal out-of-clinic radiation dosimetry in cancer patients treated with radionuclide pharmaceuticals, which can inform accurate personalized dosing of tumor(s) while safeguarding organs at risk against toxicity.
Digital Oncology: Future Targets and Challenges for Wearables to Have Impact in Shifting Patient Outcomes
Likewise, adoption and compliance (potentially lifelong) may be needed to substantially affect cancer outcomes as much as, for example, annual pan-cancer liquid biopsies. Focus on specific at-risk populations will likely demonstrate whether robust community engagement can overcome obstacles in the cancer-prevention sector for these tools. In contrast, post-diagnosis monitoring has fewer obstacles:
- There is a fixed start point in time of treatment course.
- Patient compliance may be increased due to the proximity of the event.
- Window of opportunity to mitigate the advent of complications and unplanned health encounters (e.g., proximal, late effects).
- More objective measures of the impact that treatment regimens can have on individual cancer patients.
Leveraging multiple metrics that can be acquired and combined with current wearable tools is an unmet need in the field. Digital health studies typically focus on deriving a signal and its subsequent deviation from established norms of patient activity (often coupled to electronic PROs) as a proof of concept. This has kept uses in oncology at a minimum to date. The wearables marketplace currently narrows development of devices toward singular assessments, introducing practical hurdles into any workflow design that seeks to obtain correlative metrics for an individual derived from multiple digital health approaches. These hurdles include patient compliance and burden, device interoperability, and analytic robustness. Paradoxically, AI/ML approaches may suggest predictive correlatives for cancer patient responses to treatment or risk of adverse events by retrospective analysis of data collected from multiple sources, including wearables. However, Digital Oncology as a field currently struggles with how to operationalize the validation of multiple metrics.
Possible solutions include piggybacking wearables onto prospective clinical trials as secondary endpoints. This can have the added value of secondary analysis to reveal unanticipated data characteristics. Moreover, initial results can be coupled to post-trial real-world data or evidence downstream. The tools are portable and continuously evolving and could thus be expected to continue generating data (both similar and novel) from patients outside of the confines of a clinical trial. As the evidence pool is built up from these studies, the technologies will become more useful in the assessment of surrogate endpoints. Further, new combinations of wearables, or other out-of-clinic monitoring approaches, would have the potential to build digital phenotypes for distinct patient populations and, ultimately, to pave the way for more ubiquitous, personalized Digital Oncology.
Identifying areas for which there is a conceivable path forward for high-impact personalized Digital Oncology is paramount. The list below offers examples of clinical areas in oncology where personalized digital tools could be of utility. We view these from the perspective of clear impact in the clinic or clinical research as well as an understanding of both current and future wearable devices (e.g., Grade III). However, devices are only part of the picture. Other challenges exist for Digital Oncology to be fully adopted within oncology practice or to shift patient outcomes.
- Decentralized trials with cancer patients in remote or underserved communities.
- Objective understanding, early mitigation, and long-term monitoring of adverse events or side effects (e.g., organ toxicity, cytokine release syndrome, chemotherapy- or radiotherapy-induced peripheral neuropathy (CIPN/RIPN), fatigue, autoimmunities).
- Symptom management and cancer survivor monitoring.
- Adaptive clinical trials (Proactive Patient Stratification) incorporating novel digital endpoints or surrogate markers.
- Complementing other assays or validated biomarkers in measuring response to therapy, predictive modeling, or prognosticating response.
Despite the ever-increasing technologies and infrastructure associated with wearables, Digital Oncology as a field is still in its infancy. Out-of-clinic monitoring is an area ripe for these technologies to positively impact cancer patient outcomes, and opportunities exist for more robust incorporation into current, or developing, precision medicine strategies. A coordinated effort is needed to build a viable path toward integration and adoption of these devices into routine clinical care.
Sensor-Based Frailty Assessment in Survivors of Childhood Cancer: A Pilot Study.